HYDROGEN AND OUR ENERGY FUTURE
U.S. DEPARTMENT OF ENERGY HYDROGEN PROGRAM
Hydrogen – An Overview
Hydrogen can power cars, trucks, buses, and other vehicles, as well as homes, offices, factories, and even portable electronic equipment, such as laptop computers.
What is Hydrogen?
Hydrogen, chemical symbol H, is the simplest element on earth. An atom of hydrogen has only one proton and one electron. Hydrogen gas is a diatomic molecule—each molecule has two atoms of hydrogen (which is why pure hydrogen is commonly expressed as “H2”). At standard temperature and pressure, hydrogen exists as a gas. It is colorless, odorless, tasteless, and lighter than air.
Like electricity, hydrogen is an energy carrier (not an energy source), meaning it can store and deliver energy in an easily usable form. Although abundant on earth as an element, hydrogen combines readily with other elements and is almost always found as part of some other substance, such as water (H2O), or hydrocarbons like natural gas (which consists primarily of methane, with the chemical formula, CH4). Hydrogen is also found in biomass, which includes all plants and animals.
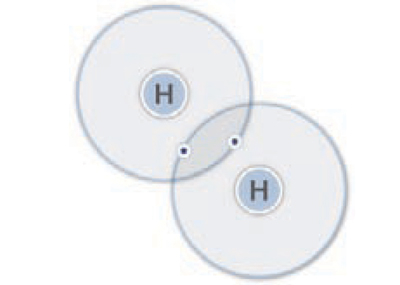
Hydrogen molecule (H2)
Basics
Although hydrogen is the most abundant element in the universe, it does not naturally exist in its elemental form on Earth. Pure hydrogen must be produced from other hydrogen containing compounds, such as fossil fuels, biomass, or water. Each production method requires a source of energy, i.e., thermal (heat), electrolytic (electricity), or photolytic (light) energy. Researchers are developing a wide range of technologies to produce hydrogen in economical, environmentally friendly ways so that we will not need to rely on any one energy resource. The great potential for diversity of supply is an important reason why hydrogen is such a promising energy carrier.
The overall challenge to hydrogen production is cost reduction. For transportation, hydrogen must be cost-competitive with conventional fuels and technologies on a per-mile basis to succeed in the commercial marketplace. This means that the cost of hydrogen (which includes the cost of production as well as delivery to the point of use) should be between $2.00 -$3.00 per gallon gasoline equivalent (untaxed).
Coal
Coal is an abundant and relatively inexpensive domestic resource. The United States has more proven coal reserves than any other country in the world—about half of the electricity produced in the United States today is generated from coal. It is important to note that hydrogen can be produced directly from coal via gasification, rather than by using coal generated electricity to produce hydrogen. The carbon dioxide created as a byproduct of coal gasification can be captured and sequestered so that the process results in near-zero greenhouse gas emissions. Coal gasification research, development, and demonstration activities seek to ensure that coal can produce clean, affordable, reliable and efficient electricity and hydrogen in the future (see below).
FutureGen
Announced in February 2003, FutureGen is a $1 billion, 10-year initiative to demonstrate the world’s first coal-based, near zero atmospheric emissions power plant to produce electricity and hydrogen. This DOE effort, although not part of the Hydrogen Fuel Initiative, supports program goals through its objective to establish the technical and economic feasibility of co-producing sequestering the carbon dioxide. FutureGen will use gasification technology to produce hydrogen directly, which is a more efficient process for the centralized production of hydrogen than coal-to-electricity-to-hydrogen generation.
www.fossil.energy.gov/futuregen

Renewable Liquid Fuel Reforming
Renewable liquid fuels, such as ethanol or bio-oils made from biomass resources, can be reformed to produce hydrogen in a distributed system—at refueling stations or stationary power sites. Biomass resources, when converted to ethanol, bio-oils, or other liquid fuels, can be transported at relatively low cost to the point of use and reformed onsite to produce hydrogen.
Reforming renewable liquids is similar to reforming natural gas. The liquid fuel is reacted with steam at high temperatures in the presence of a catalyst to produce a reformate gas composed of mostly hydrogen and carbon monoxide. Reacting the carbon monoxide with steam in a water-gas shift reaction produces additional hydrogen and carbon dioxide.
Since renewable liquids consist of larger molecules with more carbon atoms, they can be somewhat more difficult to reform than the methane in natural gas. Better catalysts will improve hydrogen yields and selectivity. And like natural gas reforming, operation, maintenance and capital equipment costs must be reduced and the energy efficiency must be improved for the hydrogen produced to be cost-competitive with conventional fuels.
Making Liquid Fuels from Biomass
Ethanol is made by converting the starch in corn into sugars and fermenting the sugars to produce ethanol. Sugars can be extracted from other biomass resources like crop or forest residues through a process involving mild acid or steam and enzyme digestion. These sugars are then fermented, producing ethanol.
Bio-oil is an oil-like liquid similar to diesel fuel. When treated with heat, biomass breaks down to produce a bio-oil.
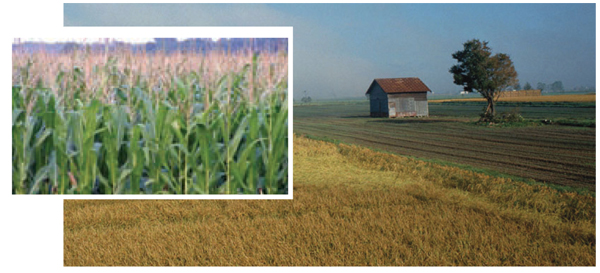
Delivery
The widespread use of hydrogen will require a national infrastructure for transportation, distribution, and delivery.
Basics
Infrastructure is required to move hydrogen from the point of production to the dispenser at a refueling station or stationary power site. Options and trade-offs for hydrogen delivery from central, semi-central, and distributed production facilities to the point of use are complex—the choice of a hydrogen production strategy greatly affects the cost and method of delivery. For example, larger, centralized facilities can produce hydrogen at relatively low costs due to economies of scale, but the delivery costs are high because the point of use is farther away. In comparison, distributed production facilities have relatively low delivery costs, but the hydrogen production costs are likely to be higher—lower volume production means higher equipment costs on a per-unit-of-hydrogen basis.
Hydrogen has been used in industrial applications for decades, but today’s delivery infrastructure and technology are not sufficient to support widespread consumer use of hydrogen. Because hydrogen has a relatively low volumetric energy density, its transportation, storage, and final delivery to the point of use comprise a significant cost and results in some of the energy inefficiencies associated with using it as an energy carrier. Key challenges to hydrogen delivery include reducing delivery cost, increasing energy efficiency, maintaining hydrogen purity, and minimizing hydrogen leakage. Further research is needed to analyze the trade-offs between hydrogen production and delivery options taken together as a system. Building a national hydrogen delivery infrastructure is a big challenge.
It will take time to develop and will likely include combinations of various technologies. Delivery infrastructure needs and resources will vary by region and type of market (e.g., urban, interstate, or rural). Infrastructure options will also evolve as the demand for hydrogen grows and as delivery technologies develop and improve.
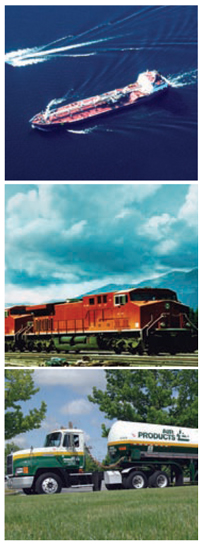
Hydrogen is delivered by barge, rail, and truck.
Pipelines
There are approximately 700 miles of hydrogen pipelines operating in the United States (compared to more than one million miles of natural gas pipelines). Owned by merchant hydrogen producers, these pipelines are located where large hydrogen refineries and chemical plants are concentrated (for example, in the Gulf Coast region).
Transporting gaseous hydrogen via existing pipelines is currently the lowest-cost option for delivering large volumes of hydrogen, but the high initial capital cost of new pipeline construction constitutes a major barrier to expanding hydrogen pipeline delivery infrastructure. Research is focused on overcoming technical concerns related to pipeline transmission, including the potential for hydrogen to embrittle the pipeline steel and welds; the need to control hydrogen permeation and leaks; and the need for lower cost, more reliable, and more durable hydrogen compression technology.
One possibility for rapidly expanding the hydrogen delivery infrastructure is to adapt part of the natural gas delivery infrastructure. Converting natural gas pipelines to carry a blend of natural gas and hydrogen (up to about 20% hydrogen) may require only modest modifications to the pipeline; converting existing natural gas pipelines to deliver pure hydrogen may require more substantial modifications.
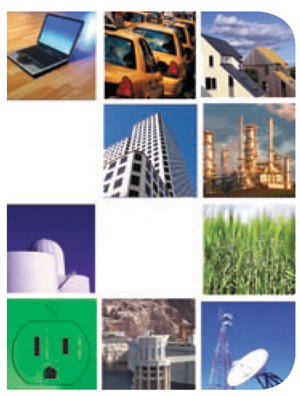
Another possible delivery process involves producing a liquid hydrogen “carrier,” such as ethanol, at a central location, pumping it through pipelines to distributed refueling stations, and processing it at the station to produce hydrogen for dispensing. Liquid hydrogen carriers offer the potential of using existing pipeline and truck infrastructure technology for hydrogen transport.
Refueling a vehicle with hydrogen is very similar to refueling a vehicle with compressed natural gas (CNG). Like CNG refueling systems, hydrogen vehicle refueling is a closed-loop system. The dispensing nozzle must “lock on” to the vehicle receptacle before any hydrogen can flow. Hydrogen dispensers are also equipped with safety devices including breakaway hoses, leak detection sensors, and grounding mechanisms. These controls provide additional safety measures in the case of human error, such as a driver trying to drive away while the dispenser is still connected to the vehicle. In today’s demonstration programs, most hydrogen vehicles are refueled by trained personnel. This will become less common as the number of demonstrations increases and hydrogen vehicles become available to consumers.

Refueling a hydrogen vehicle is similar to refueling a natural gas vehicle. Photo courtesy of Daimler Chrysler
Storage
Compact on-board hydrogen storage systems for light-duty vehicles are critical to the widespread use of hydrogen as an energy carrier. Vehicles must carry enough hydrogen to travel more than 300 miles between refuelings, without compromising vehicle cargo capacity or passenger space.
Basics
Hydrogen has the highest energy content per unit weight—but not per unit volume—of any fuel. Its relatively low volumetric energy content poses a significant challenge for storage.
Under most scenarios, stationary hydrogen storage systems have less stringent requirements than vehicular storage. Stationary systems can occupy a relatively large area, operate at higher temperatures, and compensate for slower refueling times with larger storage systems. Storage systems for vehicles, however, face much tougher challenges. They must operate within the size and weight constraints of the vehicle, enable a driving range of more than 300 miles (generally regarded as the minimum for widespread driver acceptance based on the performance of today’s gasoline vehicles), and refuel at near room temperature and at a rate fast enough to meet drivers’ requirements (generally only a few minutes). Because of these challenges, hydrogen storage research is focused primarily on vehicular applications.
Many consider on-board hydrogen storage to be the greatest technical challenge to widespread commercialization of hydrogen fuel cell vehicles. Current approaches (see below) include compressed hydrogen gas tanks, liquid hydrogen tanks, and hydrogen storage in materials. Researchers in government, industry, and academia are working toward technical targets specific to the entire storage system—which includes the tank, storage media, valves, regulators, piping, added cooling capacity, and other “balance-of-plant components.” Research is focused on improving the weight, volume (gravimetric and volumetric capacity), and cost of current hydrogen storage systems, as well as identifying and developing new technologies that can achieve similar performance, and at a similar cost, as gasoline fuel storage systems.
Application and Use
Hydrogen can be converted into usable energy through fuel cells or by combustion in turbines and engines. Fuel cells now in development will not only provide a new way to produce power, but will also significantly improve energy conversion efficiency, especially in transportation applications.
Overview
Hydrogen is a versatile energy carrier that can be used to power nearly every end-use energy need. The fuel cell—an energy conversion device that can efficiently convert and use the power of hydrogen—is key to making it happen. Stationary fuel cells can be used for backup power, power for remote locations, distributed power generation, and cogeneration (in which excess heat released during electricity generation is used for other applications). Fuel cells can power almost any portable application that uses batteries, from hand-held devices to portable generators. Fuel cells can also provide primary and auxiliary power for transportation, including personal vehicles, trucks, buses, and marine vessels.

Photo courtesy of General Motors
Why Fuel Cells?
Fuel cells directly convert the chemical energy in hydrogen to electricity and heat. Inside a fuel cell, hydrogen electrochemically combines with oxygen from the air to create electricity, with pure water and potentially useful heat as the only byproducts.
Hydrogen-powered fuel cells are not only pollution-free, but also can have two to three times the efficiency of traditional internal combustion technologies. A conventional combustion- based power plant typically generates electricity at efficiencies of 33 to 35%, while fuel cell systems can generate electricity at efficiencies of up to 60% (and even higher with cogeneration). In normal driving, the gasoline engine in a conventional car is less than 20% efficient in converting the chemical energy in gasoline into power that moves the vehicle. Hydrogen fuel cell vehicles, which use electric motors, are much more energy efficient and use up to 60% of the fuel’s energy. This corresponds to more than a 50% reduction in fuel consumption, compared to a conventional vehicle with a gasoline internal combustion engine.* In addition, fuel cells operate quietly, have fewer moving parts, and are well suited to a variety of applications.
*U.S. Department of Energy, Energy Information Administration, Supplemental tables to the Annual Energy Outlook 2005, “Transportation Demand Sector,” Table 47, (2005), 45.
Fuel Cell Basics
A single fuel cell consists of an electrolyte sandwiched between two electrodes, an anode and a cathode. The power produced by a fuel cell depends on several factors, including the fuel cell type, size, temperature at which it operates, and pressure at which gases are supplied to the cell. A single fuel cell produces 1 volt of electricity or less. To generate more electricity, individual fuel cells are combined in a series to form a stack. (The term “fuel cell” is often used to refer to the entire stack, as well as to the individual cell). Depending on the application, a fuel cell stack may contain hundreds of individual cells layered together. This “scalability” makes fuel cells ideal for a wide variety of applications, from laptop computers (50-100 Watts) to homes (1-5kW), vehicles (50-125 kW), and central power generation (1-200 MW or more).
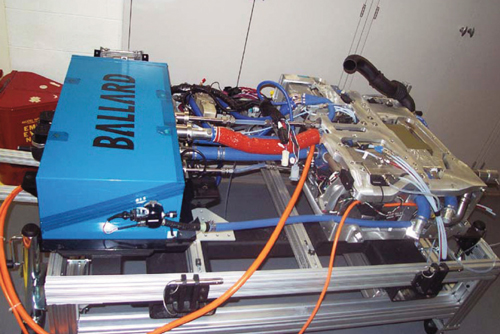
Challenges
Reducing cost and improving durability are the two most significant challenges to fuel cell commercialization. Fuel cell systems must be cost-competitive with, and perform as well or better than, traditional power technologies over the life of the system.
Materials and manufacturing costs are currently too high for catalysts, bipolar plates, membranes, and gas diffusion layers. Today, the costs for automotive internal combustion engine power plants are about $25-$35/kW; for transportation applications, a fuel cell system needs to cost approximately $30/kW (at volumes of 500,000 units per year) for the technology to be competitive. For stationary systems, the acceptable price point is considerably higher – $400-$750/kW is considered necessary for widespread commercialization ($1,000/kW for initial “early adopter” applications). (Data current as of March 2007. For the most up-to-date numbers, please refer to the Hydrogen Program Multi-Year Research, Development, and Demonstration Plan www.eere.energy. gov/hydrogenandfuelcells/mypp/)
In addition to cost-related challenges, adequate fuel cell system durability has not been established. To be considered ready for widespread use in transportation, fuel cell power systems must demonstrate durability and reliability similar to today’s automotive engines. Specifically, this means a 5,000- hour operating lifespan (the equivalent of 150,000 miles) and lithe ability to perform over the full range of dynamic vehicle operating conditions, including temperatures and climates (various degrees of humidity and ambient temperatures of - 40°C to +40°C, for example). Requirements for stationary applications are slightly different because the associated duty cycles are less demanding. To compete with other distributed power generation systems, stationary fuel cells must demonstrate more than 40,000 hours (nearly 5 years) of reliable operation in ambient temperatures of -40°C to +50°C to be considered ready for the mainstream market. For both transportation and stationary applications, research is needed to identify and develop new materials to reduce the cost and extend the life of fuel cell stack components. Low cost, high volume manufacturing processes will also help to make fuel cell systems competitive with traditional technologies.
Safety, Codes and Standards
The United States safely produces and uses more than nine million tons of hydrogen each year, primarily in controlled industrial environments. Hydrogen can be used safely in consumer environments as well, when users understand its basic properties and observe guidelines.
Hydrogen Safety
Like gasoline or natural gas, hydrogen is a fuel that must be handled appropriately. The characteristics of hydrogen are different from those of other fuels, but it can be used as safely as other common fuels when guidelines are observed.
Hydrogen has a number of properties that are advantageous with regard to safety. It is much lighter than air and has a rapid diffusivity (3.8 times faster than natural gas), which means that when released in an open environment, it disperses quickly into a non-flammable concentration. Hydrogen rises at a speed of almost 20 meters per second, twice as fast as helium and six times faster than natural gas.
With the exception of oxygen, any gas can cause asphyxiation in high enough concentrations. But because hydrogen is buoyant and disperses rapidly, it is less likely to be confined than other gases and therefore poses less risk as an asphyxiant.
Hydrogen is non-toxic and non-poisonous. It will not contaminate groundwater and is a gas under normal atmospheric conditions with a very low solubility in water. A release of hydrogen is not known to contribute to atmospheric pollution or water pollution.

Photo courtesy of CaFCP
Hydrogen is odorless, colorless, and tasteless, and thus undetectable by human senses. By comparison, natural gas is also odorless, colorless, and tasteless, but industry adds a sulfurcontaining odorant to make it readily detectable by people. Odorants are not added to hydrogen because currently, there is no known odorant light enough to “travel with” hydrogen or disperse in air at the same rate. Odorants also contaminate fuel cells. Industry considers these properties when designing structures where hydrogen is used or stored and includes redundant safety systems that include leak detection sensors and ventilation systems.
Hydrogen burns with a pale blue, nearly invisible, flame. Hydrogen flames also have low radiant heat compared to hydrocarbon flames. Detection sensors built into hydrogen systems can quickly identify any leak and eliminate the potential for undetected flames. Since hydrogen flames emit relatively low levels of heat, (the flame itself is just as hot), the risk of secondary fires by ignition of other combustibles in the vicinity is lower.
An explosion cannot occur in a tank or any contained location with only hydrogen. An oxidizer, such as oxygen, must be present. Hydrogen does burn very quickly, however, sometimes making a loud noise that can be mistaken for an explosion. Under optimal combustion conditions, the energy required to initiate hydrogen combustion is significantly lower than that required for other common fuels including natural gas or gasoline. At low concentrations of hydrogen fuel in air, the energy required to initiate combustion is similar to that of other fuels.

Codes and Standards
Hydrogen codes and standards are being developed to provide the information needed to safely build, maintain, and operate hydrogen and fuel cell systems and facilities; ensure uniformity of safety requirements; and provide local officials and safety inspectors with the information needed to certify hydrogen systems and installations. Developing codes and standards provides a systematic and accurate means of minimizing risk and ensuring public safety. Many organizations are cooperating to develop hydrogen codes and standards. Today, hydrogen components are built to meet strict manufacturer and published guidelines and undergo third-party testing for safety and structural integrity.
Ford Is A Pioneer In Hydrogen Technology
Hydrogen Powered Vehicles
Ford began working on hydrogen technology in the early 1990s. The company’s first hydrogen internal combustion engine demonstration vehicle, released in 2001, was based on a lightweight aluminum sedan body, which also was used in the development of the company’s first drivable hydrogen fuel cell vehicle.
The company currently has a fleet of 30 hydrogen-powered Focus20fuel cell vehicles on the road as part of a worldwide, seven-city program to conduct real world testing of fuel cell technology. The fleet has accumulated more than 650,000 miles since its inception. With this fleet on the ground, information is being generated in different local environmental conditions that can be integrated into future fuel cell vehicle propulsion systems.
In addition, Ford recently partnered with the Wayne County Airport Authority and the Southeast Michigan Council of Governments to deliver two Ford E-450 hydrogen-fueled buses for use at Detroit Metropolitan Airport. As travelers are shuttled between terminals on the eco-friendly buses, CO2 emissions are reduced by 99.7 percent.
Powered by a 6.8-liter V 10 internal combustion engine that runs exclusively on hydrogen fuel, the highly efficient Ford E-450 shuttle buses have near zero emissions of regulated pollutants and greenhouse gases, and are also all-weather capable.
Ford has also supplied these ultra-clean buses to sites in locations such as Orlando, Las Vegas and Canadian provinces.
“Ford is the world’s first automaker to deliver commercial vehicles powered by internal combustion engines that are fueled exclusively with hydrogen,” says Sue Cischke, senior vice president, Sustainability, Environment and Safety Engineering. “These buses represent part of our strategy and commitment to delivering transportation solutions that emit less CO2 and reduce our dependence on oil.
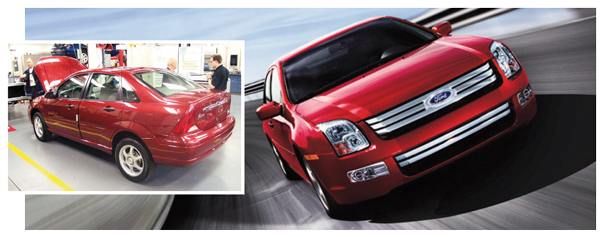
Ford Hydrogen-Powered Bus To Begin Production Soon
Michael Collins, FCN
Ford Motor Company will begin producing the world's first fleet of commercial hydrogen-powered internal combustion engine (H2ICE) vehicles this month.
Eight E-450 shuttle busses will go to tourist destinations in Florida, where they will be highly visible to the public.
"Because these buses will be in continuous service in very public places, we'll be able to communicate to hundreds of thousands of consumers that Ford is developing innovative products to meet future transportation needs," said Vance Zanardelli, chief engineer of Ford's H2ICE program.
The potential benefits of hydrogen are huge. Hydrogen could decrease dependence on foreign oil and reduce greenhouse gas emissions, improving air quality. But it faces significant challenges; there are very few hydrogen fueling stations and current storage tanks hold only enough fuel to travel 150 to 200 miles.
"We've demonstrated that hydrogen can be clean and efficient and reliable, but the biggest issue remains how to store enough hydrogen on the vehicle in a given space. The tanks are also still too expensive." said Bob Natkin, technical leader for H2 IC Engine Applications Research and Advanced Engineering.
The 12-passenger shuttle, first introduced at the 2005 North American International Auto Show, is equipped with a 30 gallon equivalent, 5,000 pounds-per-square-inch hydrogen fuel tank. It's propelled by a re-engineered version of Ford's 6.8-liter V 10 engine, which produces near-zero emissions.
Speaking at the Handelsblatt conference on automotive technologies in Munich, Gerhard Schmidt, Ford's vice president for Research and Development, said the hydrogen internal combustion engine program helps pave the way to other applications.
"The development and refinement of H2ICE and H2ICE hybrids can provide one stepping stone to the widespread use of hydrogen fuel and the introduction to fuel-cell technology," Schmidt said.
Bringing the H2ICE busses to market required extensive testing that positioned Ford ahead of its competitors, according to John Lapetz, manager, Ford Hydrogen Vehicle Program.
"By putting ourselves through the trials of coming up with a production-ready vehicle, we have learned what would have never learned by simply doing research," said Lapetz. "We've solved some durability and extreme weather issues that have given us a real head start on those that are still doing small one-off programs."
Putting H2ICE busses on the road is only part of the picture, according to Zanardelli. Ford's H2ICE research is drawing attention from universities and national laboratories in the U.S. and Europe, he added.
"They perceive Ford as clearly in a leadership role," he said. "It's a good position to be in, and we intend to keep it that way."
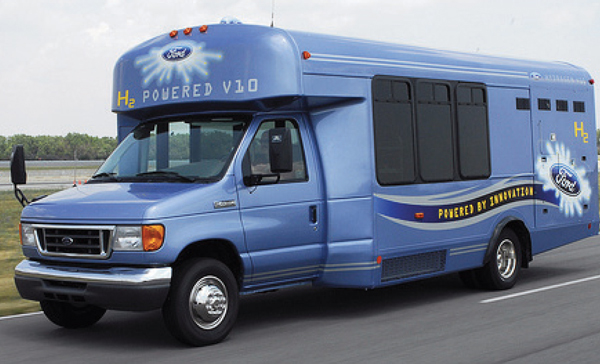
© 2015 TLC Magazine Online, Inc. |